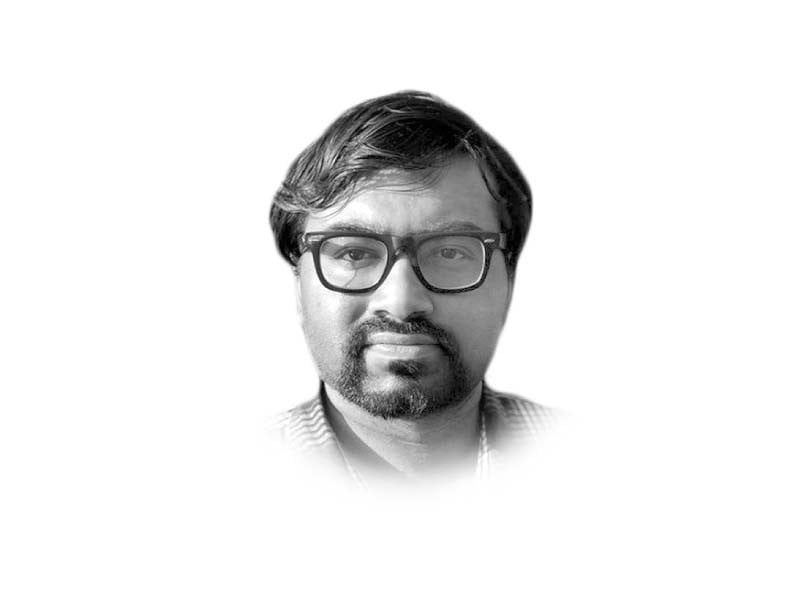
The lives of all stars are at the mercy of the interplay between two forces, those being the inward pull of gravity and the outward push of radiation. However in the case that one of these forces overcomes the other, the consequences for the star in question can be intriguing to say the least. This becomes especially pertinent once a star has exhausted the fuel (i.e. hydrogen) in its core. With nuclear fusion coming to a halt we can expect the star to succumb to the will of gravity as a result of which it will begin to collapse. In the case of “small” stars like our Sun, the star is expected to become a rather peculiar object known as a white dwarf.
On his voyage to England from what was then Madras in British India, in 1930, a young physicist by the name of Subrahmanyan Chandrasekhar pondered upon the formation of these white dwarfs. By considering the effects of gravity and at the time, cutting edge laws of quantum mechanics, he came upon a novel result whilst at sea. He found that nature had placed a maximum mass limit on white dwarfs, so as to prevent the object from violating the laws of quantum mechanics. Known as the Chandrasekhar mass limit, it was eventually verified by scores of white dwarf observations conducted by many astronomers subsequently.
Nature does not produce a white dwarf greater than 1.44 times the mass of the Sun. Furthermore according to Chandrasekhar’s calculations the size of a white dwarf would be almost that of the Earth. Imagine stuffing the Sun’s equivalent of mass inside the confines of the Earth. Such an exotic object would be incredibly dense, so that a morsel of white dwarf matter would weigh over a dozen tons! The gravity of a white dwarf is also enormously intense, almost a million times stronger than that of the Earth’s. Such a state is only achievable due to the progenitor star’s mass. The gravity of the star compresses the atoms in its core until the electrons within those atoms are tightly packed alongside one another.
Recall that the Chandrasekhar mass limit is a consequence of the laws of quantum mechanics that govern the realm of atoms and subatomic particles. Since electrons must occupy distinct “states” they resist any attempt to be forced together, resulting in a new opposing force known as “degeneracy pressure”. Thus as long as a white dwarf remains within the Chandrasekhar mass limit, it can continue to exist in a stable manner, as the degeneracy pressure of the electrons will continue to resist the inward pull of gravity.
Perhaps at this point you may be contemplating that if fusion has ceased altogether within a white dwarf, then that would imply that such an object will produce no light at all, and therefore should not be visible. But then how have astronomers managed to observe them in the first place?
It is indeed true that a white dwarf does not produce any light of its own, however since it was once the core of a star, it does possess some leftover radiation that is still “trapped” inside. This makes white dwarfs generally much less luminous than stars that are still burning hydrogen and other elements in their cores. For instance the star Sirius has a smaller white dwarf companion in orbit around it known as Sirius B. The brightness of Sirius B is about a thousand times less than that of Sirius! Due to the extremely high densities inside white dwarfs, the leftover light continuously bounces around until it finally reaches the surface from which it can escape out into the cosmos.
White dwarfs over the course of their lifetime will continue to lose all of the leftover light until it is completely snuffed out. There are some other fascinating aspects of white dwarfs worth considering. One of them being that if present in a binary star system (i.e. two stars bound together), the white dwarfs’ stronger gravity can siphon material off from its stellar companion. This process known as accretion can result in the white dwarf becoming brighter by several orders of magnitude as the material is heated up to temperatures of millions of degrees Kelvin. This phenomenon is known as a classical nova, of which several hundred exist in our galaxy and beyond.
The classical nova scenario raises another perplexing question. If a white dwarf continues to gather more and more material, what exactly would transpire once its total mass has exceeded the Chandrasekhar limit? The limit as we discussed earlier forbids the violation of the laws of quantum mechanics. Clearly we can infer that the white dwarf will cease to remain stable as the degeneracy pressure will be unable to resist the now even greater pull of gravity thus triggering a rapid collapse, which causes one final burst of nuclear fusion. It is in such conditions that elements heavier than iron are forged. This collapse along with the energy released by the burst of fusion results in the white dwarf undergoing something radical. It explodes, in an event known as a type 1a supernova. The light that emanates from such an explosion exceeds the total luminous output of an entire galaxy!
It would not be wrong to remark that within any white dwarf resides the equivalent energy of a billion suns, waiting to be unleashed for the entire universe to marvel at.
Published in The Express Tribune, May 9th, 2024.
Like Opinion & Editorial on Facebook, follow @ETOpEd on Twitter to receive all updates on all our daily pieces.
COMMENTS
Comments are moderated and generally will be posted if they are on-topic and not abusive.
For more information, please see our Comments FAQ